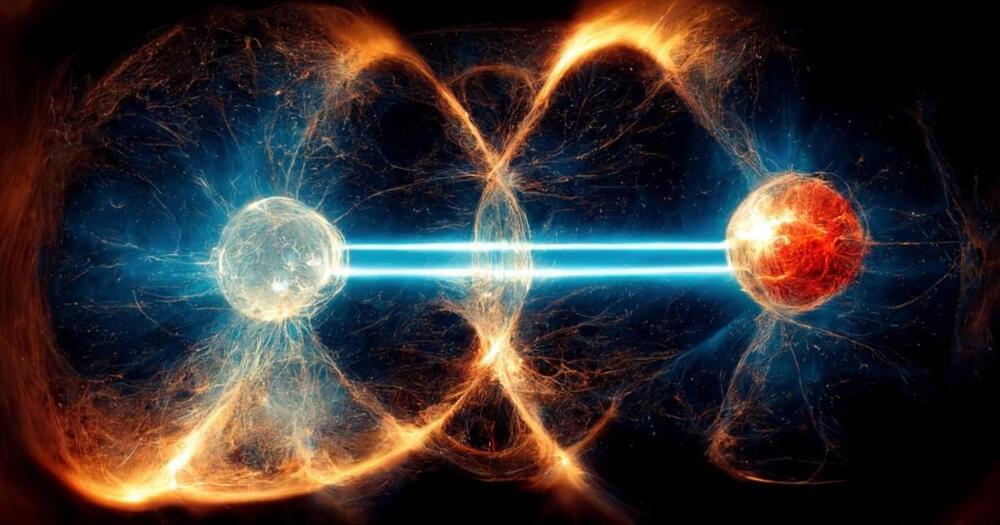
For the first time, an experiment was able to demonstrate that it isn’t just identical quantum particles that can become entangled, but particles with opposite electric charges, too. (The π+ and the π–, for what it’s worth, are one another’s antiparticle.) The technique of passing two heavy nuclei very close to one another at nearly the speed of light allows for photons, arising from the electromagnetic field of each nucleus, to interact with the other nucleus, occasionally forming a rho particle that decays into two pions. When both nuclei do this at once, the entanglement can be seen, and the radius of the atomic nucleus can be measured.
It’s also remarkable that measuring the size of the nucleus through this method, which uses the strong force rather than the electromagnetic force, gives a different, larger result than one would get by using the nuclear charge radius. As lead author on the study, James Brandenburg, put it, “Now we can take a picture where we can really distinguish the density of gluons at a given angle and radius. The images are so precise that we can even start to see the difference between where the protons are and where the neutrons are laid out inside these big nuclei.” We now have a promising method to probe the internal structure of these complex, heavy nuclei, with more applications, no doubt, soon to come.