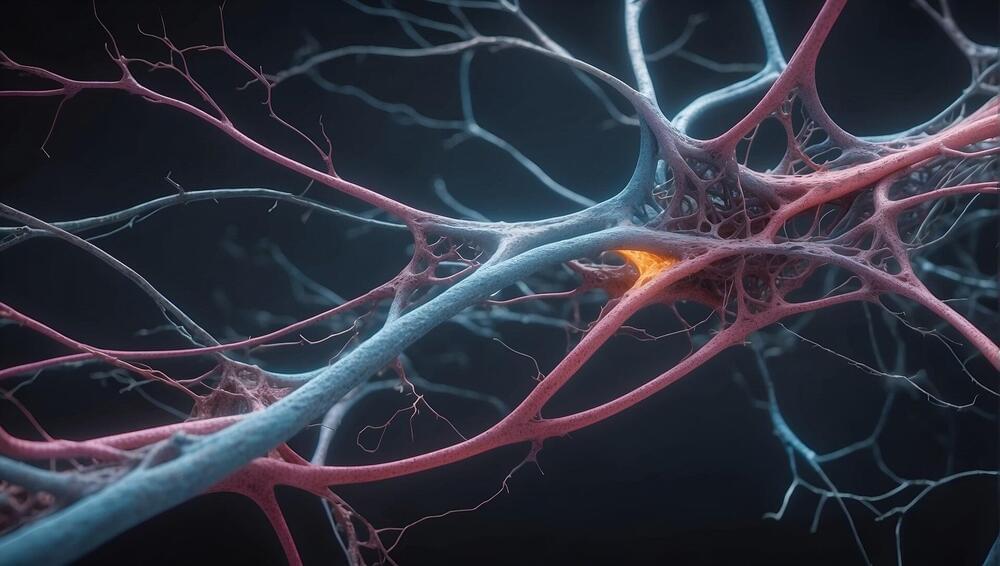
Salt, or more precisely the sodium it contains, is very much a “Goldilocks” nutrient. Low sodium levels cause a drop in blood volume, which can have serious, sometimes deadly, health consequences. Conversely, too much salt can lead to high blood pressure and cardiovascular disease.
In modern America, where most people consume a high-salt diet, almost no one is in danger of having too little salt. However, given the critical importance of sodium for body and brain functions, evolution has developed a powerful drive to consume salt in situations where there is a deficiency.
Understanding the brain circuitry that controls salt appetite has proved elusive, but now a new study by University of Iowa researchers has identified the first and, thus far, only neurons necessary for salt appetite.