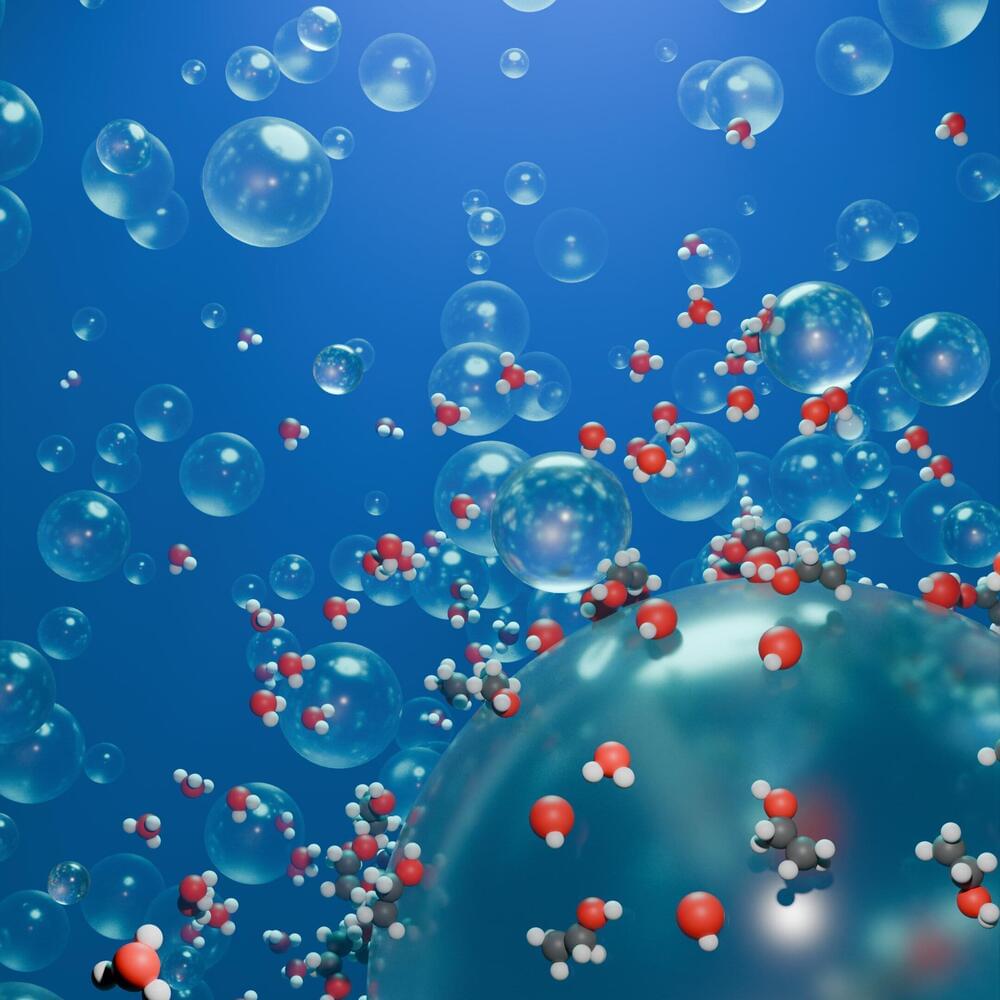
Gases are essential for many chemical reactions, and bubbles are one way for these gases to be held in solution. When compared to larger bubbles, nanobubbles have increased stability—meaning that they can remain in a solution longer without popping. Due to their increased stability, they allow for higher availability of gases in solution, allowing more time for chemical reactions to occur.
Led by Dr. Hamidreza Samouei, researchers at Texas A&M University are advancing their understanding of what makes nanobubbles—bubbles with diameters smaller than a single strand of hair—so stable and what factors play a role in their stability. Their findings appear in a recent issue of The Journal of Physical Chemistry.
“When we inject gas at the industrial scale, we don’t want to waste that gas. We want to maximize its use for chemical reactions,” said Samouei, a research assistant professor in the Harold Vance Department of Petroleum Engineering. “That’s the main purpose, to keep the gas in solution for a very, very long time, ideally infinite time; to keep the gas in solution without bursting.”