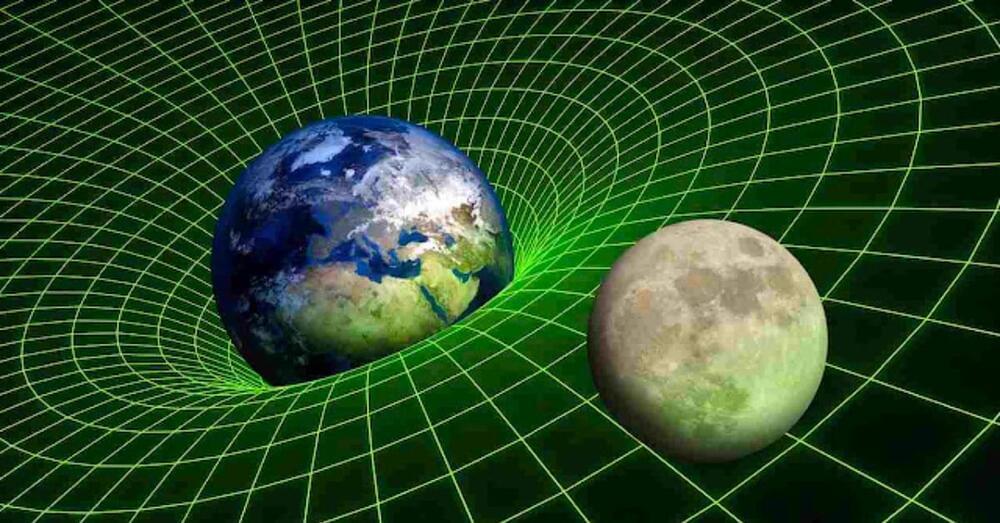
An international team of astrophysicists has made a puzzling discovery while analyzing certain star clusters. The University of Bonn played a major role in the study. The finding challenges Newton’s laws of gravity, the researchers write in their publication. Instead, the observations are consistent with the predictions of an alternative theory of gravity. However, this is controversial among experts. The results have now been published in the Monthly Notices of the Royal Astronomical Society.
In their work, the researchers investigated the so-called open star clusters. These are formed when thousands of stars are born within a short time in a huge gas cloud. As they “ignite,” the galactic newcomers blow away the remnants of the gas cloud. In the process, the cluster expands considerably. This creates a loose formation of several dozen to several thousand stars. The weak gravitational forces acting between them hold the cluster together.
“In most cases, open star clusters survive only a few hundred million years before they dissolve,” explains Prof. Dr. Pavel Kroupa of the Helmholtz Institute of Radiation and Nuclear Physics at the University of Bonn. In the process, they regularly lose stars, which accumulate in two so-called “tidal tails.” One of these tails is pulled behind the cluster as it travels through space. The other, in contrast, takes the lead like a spearhead.