Archive for the ‘bioengineering’ category: Page 126
Sep 26, 2019
Meet Eight Tech Titans Investing In Synthetic Biology
Posted by Genevieve Klien in categories: bioengineering, biotech/medical, computing, food, sustainability
“DNA is like a computer program but far, far more advanced than any software ever created.” Bill Gates wrote this in 1995, long before synthetic biology – a scientific discipline focused on reading, writing, and editing DNA – was being harnessed to program living cells. Today, the cost to order a custom DNA sequence has fallen faster than Moore’s law; perhaps that’s why the Microsoft founder is turning a significant part of his attention, and wallet, towards this exciting field.
Bill Gates is not the only tech founder billionaire that sees a parallel between bits and biology, either. Many other tech founders – the same people that made their money programming 1s and 0s – are now investing in biotech founders poised to make their own fortunes by programming A’s, T’s, G’s and C’s.
The industry has raised more than $12.3B in the last 10 years and last year, 98 synthetic biology companies collectively raised $3.8 billion, compared to just under $400 million total invested less than a decade ago. Synthetic biology companies are disrupting nearly every industry, from agriculture to medicine to cell-based meats. Engineered microorganisms are even being used to produce more sustainable fabrics and manufacture biofuels from recycled carbon emissions.
Sep 26, 2019
Can We Redesign The Modern City With Synthetic Biology? Could We Grow Our Houses Instead Of Building Them?
Posted by Klaus Baldauf in categories: bioengineering, biotech/medical, food, habitats, sustainability
Imagine waking up every morning in a house that is just as alive as you are. With synthetic biology, your future home could be a living, breathing marvel of nature and biotechnology. Yes, it’s a bold ambition. But this kind of visionary thinking could be the key to achieving sustainability for modern cities.
Our current homes and cities are severely outdated. Dr. Rachel Armstrong, a synthetic biologist and experimental architect, says, “All our current buildings have something in common: they’re built using Victorian technologies.” Traditional design, manufacturing, and construction processes demand huge amounts of energy and resources, but the resulting buildings give nothing back. To make our future sustainable, we need dynamic structures that give as much as they take. We need to build with nature, not against it.
In nature, everything is connected. For the world’s tallest trees—the California redwoods— their lives depend on their connection to each other as well as on a host of symbiotic organisms. Winds and rain batter the California coast, so redwoods weave their roots together for stability, creating networks that can stretch hundreds of miles. The rains also leach nutrients from the soil. But fungi fill the shortage by breaking down dead organic matter into food for the living. A secondary network of mycelia—the root-like structures of the fungi—entwine with the tree roots to transport nutrients, water, and chemical communications throughout the forest. What if our future cities functioned like these symbiotic networks? What if our future homes were alive?
Sep 26, 2019
Human Embryoid Research! — Dr. Deborah Gumucio, Ph.D — University of Michigan — ideaXme — Ira Pastor
Posted by Ira S. Pastor in categories: 3D printing, aging, bioengineering, bioprinting, biotech/medical, complex systems, DNA, genetics, health, transhumanism
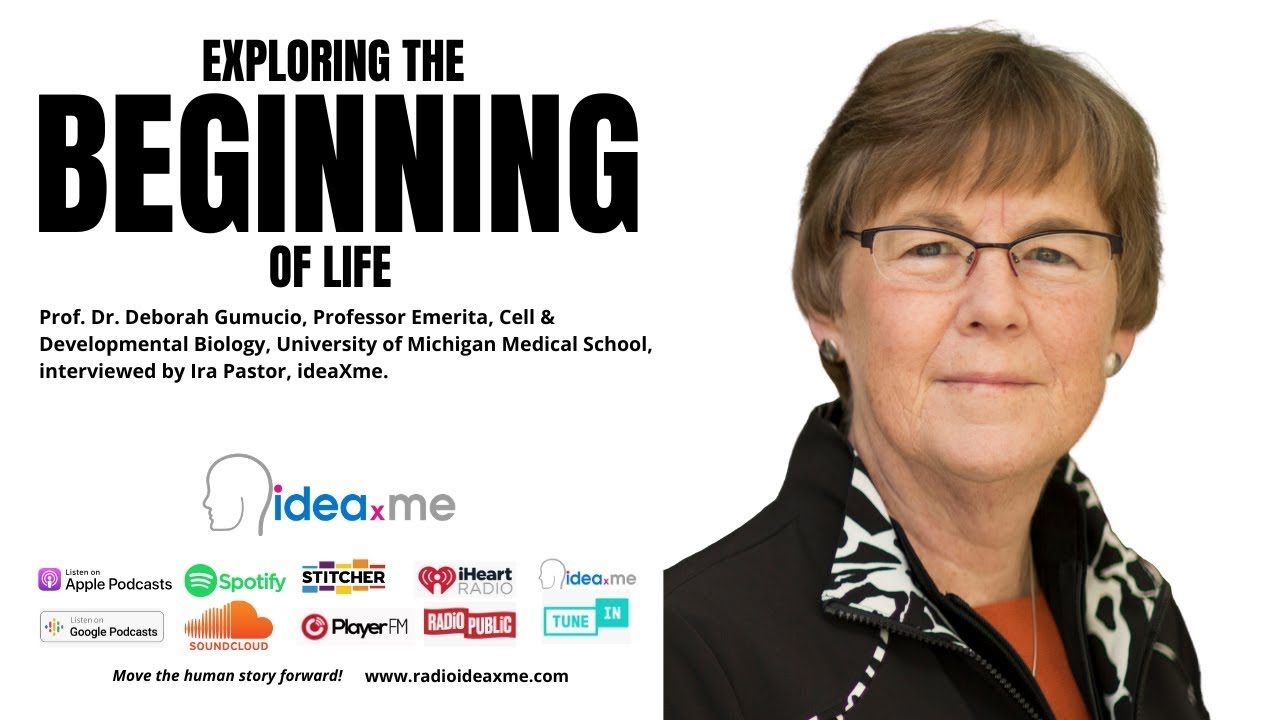
Sep 22, 2019
With Food Security Becoming One Of Our Biggest Challenges For Humankind’s Survival, What’s On The Menu For The 9 Billion People Inhabiting The World By 2050?
Posted by Genevieve Klien in categories: bioengineering, biological, climatology, security
Food security is one of the biggest challenges we’re facing as we move further into this century. Changing climate, pests, stress on water and land are all limiting our ability to produce sufficient amounts of food. making food production an issue.
Synthetic biology offers ways to help produce and supply enough safe and nutritious food sustainably for the estimated 9 billion people that will inhabit the planet by 2050.
Here are a few ways how.
Sep 19, 2019
The design, construction and characterization of new nanovibrational bioreactors for osteogenesis
Posted by Gerard Bain in categories: bioengineering, biotech/medical, computing, life extension, nanotechnology
In regenerative medicine, scientists aim to significantly advance techniques that can control stem cell lineage commitment. For example, mechanical stimulation of mesenchymal stem cells (MSCs) at the nanoscale can activate mechanotransduction pathways to stimulate osteogenesis (bone development) in 2-D and 3D culture. Such work can revolutionize bone graft procedures by creating graft material from autologous or allogenic sources of MSCs without chemically inducing the phenomenon. Due to increasing biomedical interest in such mechanical stimulation of cells for clinical use, both researchers and clinicians require a scalable bioreactor system to provide consistently reproducible results. In a new study now published on Scientific Reports, Paul Campsie and a team of multidisciplinary researchers at the departments of biomedical engineering, computing, physics, and molecular, cell and systems biology engineered a new bioreactor system to meet the existing requirements.
The new instrument contained a vibration plate for bioreactions, calibrated and optimized for nanometer vibrations at 1 kHz, a power supply unit to generate a 30 nm vibration amplitude and custom six-well cultureware for cell growth. The cultureware contained magnetic inserts to attach to the bioreactor’s magnetic vibration plate. They assessed osteogenic protein expression to confirm the differentiation of MSCs after initial biological experiments within the system. Campsie et al. conducted atomic force microscopy (AFM) of the 3D gel constructs to verify that strain hardening of the gel did not occur during vibrational stimulation. The results confirmed cell differentiation to be the result of nano-vibrational stimulations provided by the bioreactor alone.
The increasing incidence of skeletal injuries due to age-related conditions such as osteoporosis and osteoarthritis is a metric of the depleting quality of human life. The development of treatments for increased bone density or fracture healing are prime targets for the regenerative potential of mesenchymal stem cells (MSCs). Researchers have demonstrated controlled osteogenesis (development of bones) of MSCs via mechanical stimulation using several methods, including passive and active strategies. Passive methods typically alter the substrate topography to influence the cell adhesion profile, while active methods include exposure to varied forces from external sources.
Sep 17, 2019
Nano-Sized Solution for Efficient and Versatile CRISPR Gene Editing
Posted by Quinn Sena in categories: bioengineering, biotech/medical, genetics, nanotechnology
If used to make non-heritable genetic changes, CRISPR gene-editing technology holds tremendous promise for treating or curing a wide range of devastating disorders, including sickle cell disease, vision loss, and muscular dystrophy. Early efforts to deliver CRISPR-based therapies to affected tissues in a patient’s body typically have involved packing the gene-editing tools into viral vectors, which may cause unwanted immune reactions and other adverse effects.
Now, NIH-supported researchers have developed an alternative CRISPR delivery system: nanocapsules. Not only do these tiny, synthetic capsules appear to pose a lower risk of side effects, they can be precisely customized to deliver their gene-editing payloads to many different types of cells or tissues in the body, which can be extremely tough to do with a virus. Another advantage of these gene-editing nanocapsules is that they can be freeze-dried into a powder that’s easier than viral systems to transport, store, and administer at different doses.
In findings published in Nature Nanotechnology [1], researchers, led by Shaoqin Gong and Krishanu Saha, University of Wisconsin-Madison, developed the nanocapsules with specific design criteria in mind. They would need to be extremely small, about the size of a small virus, for easy entry into cells. Their surface would need to be adaptable for targeting different cell types. They also had to be highly stable in the bloodstream and yet easily degraded to release their contents once inside a cell.
Sep 16, 2019
Meet Five Synthetic Biology Companies Using AI To Engineer Biology
Posted by Klaus Baldauf in categories: bioengineering, biological, genetics, robotics/AI
TVs and radios blare that “artificial intelligence is coming,” and it will take your job and beat you at chess.
But AI is already here, and it can beat you — and the world’s best — at chess. In 2012, it was also used by Google to identify cats in YouTube videos. Today, it’s the reason Teslas have Autopilot and Netflix and Spotify seem to “read your mind.” Now, AI is changing the field of synthetic biology and how we engineer biology. It’s helping engineers design new ways to design genetic circuits — and it could leave a remarkable impact on the future of humanity through the huge investment it has been receiving ($12.3b in the last 10 years) and the markets it is disrupting.
Sep 16, 2019
The Regenerage Show- Episode #4 — “Whole Organism Rejuvenation and Combinatorial Biologics” — Ira Pastor — Host
Posted by Ira S. Pastor in categories: aging, bioengineering, biotech/medical, business, DNA, futurism, health, Peter Diamandis, science, transhumanism
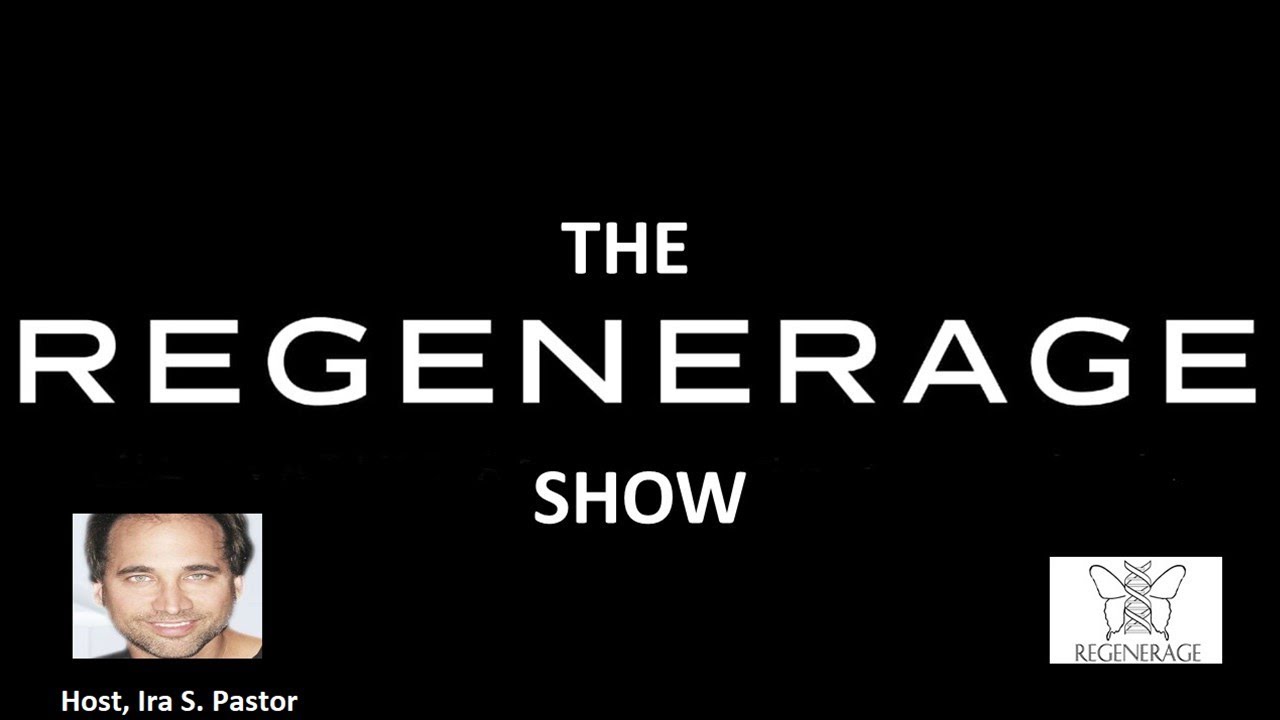
Sep 16, 2019
Researchers build microscopic biohybrid robots propelled by muscles, nerves
Posted by Genevieve Klien in categories: bioengineering, robotics/AI
Researchers have developed soft robotic devices driven by neuromuscular tissue that triggers when stimulated by light—bringing mechanical engineering one step closer to developing autonomous biobots.
In 2014, research teams led by mechanical science and engineering professor Taher Saif and bioengineering professor Rashid Bashir at the University of Illinois worked together to developed the first self-propelled biohybrid swimming and walking biobots powered by beating cardiac muscle cells derived from rats.
“Our first swimmer study successfully demonstrated that the bots, modeled after sperm cells, could in fact swim,” Saif said. “That generation of singled-tailed bots utilized cardiac tissue that beats on its own, but they could not sense the environment or make any decisions.”